Beetle Juice
Creating Water for a Drying World
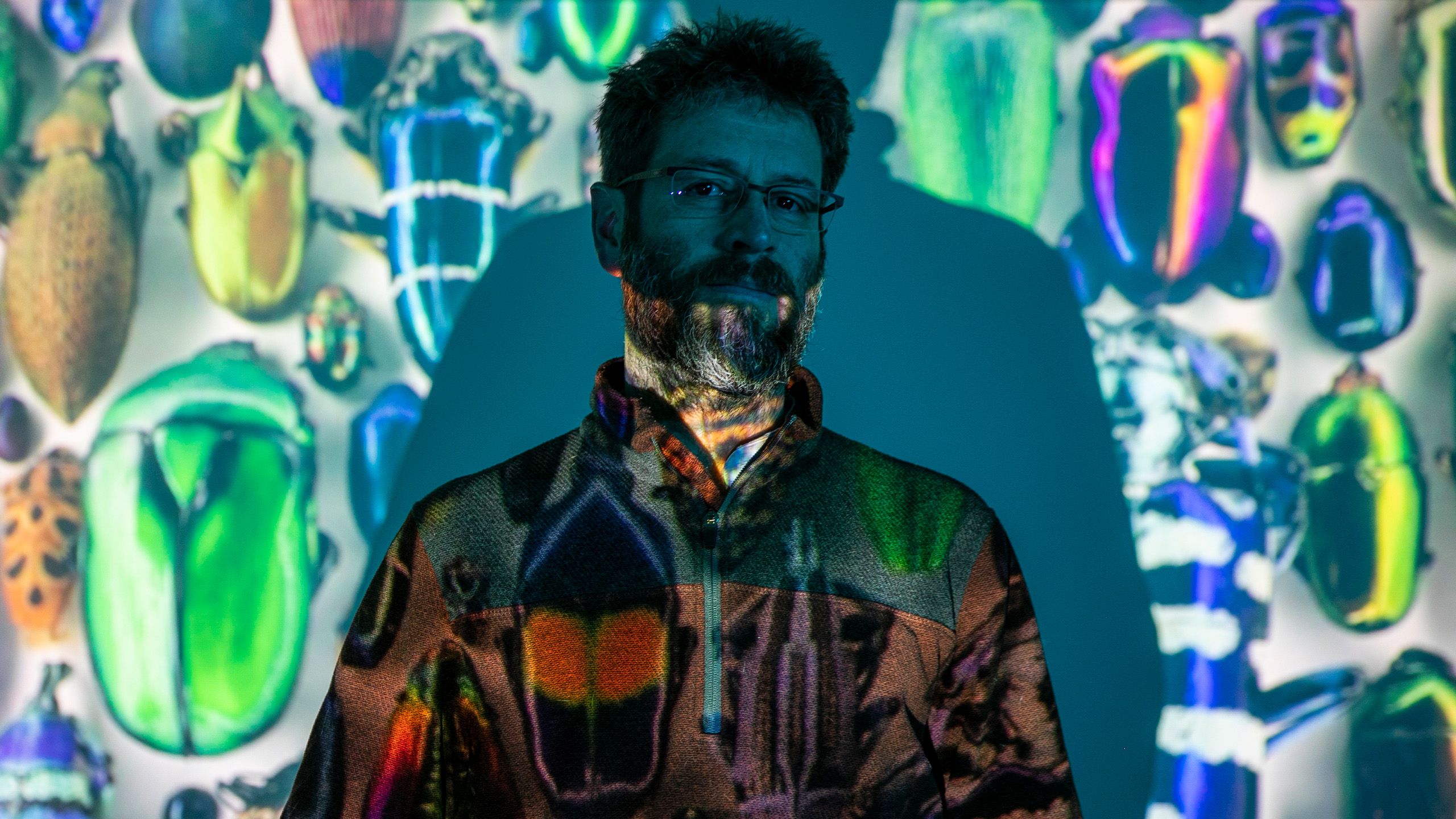
Humans have long looked to nature to solve some of the most pressing and complex design challenges. English inventor Percy Shaw patented the road stud in 1934 after he was inspired by the reflective nature of cat eyes. To develop bird–safe glass, German engineering company Arnold Glas observed Orb weaver spiders, who build their webs with ultraviolet silk. As Hunter King, assistant professor in the Department of Physics at Rutgers–Camden, works to solve the country’s growing water scarcity crisis, he’s found his muse in a South African insect no bigger than an almond.
King became interested in biomimicry—the emulation of the models, systems, and elements of nature for the purpose of solving complex human problems—while conducting postdoctoral research at Harvard University. His colleague at the time was studying the fog-harvesting abilities of the Namib desert beetle. His research, which expanded on a popularized wettability (meaning, the ability of a liquid to spread over a surface) theory, did not sit well with King.

Biomimicry is the imitation of natural biological designs or processes in engineering or invention. (Merriam-Webster.com Dictionary) (image courtesy of biomimicry.org)
Biomimicry is the imitation of natural biological designs or processes in engineering or invention. (Merriam-Webster.com Dictionary) (image courtesy of biomimicry.org)

“When they cared about wettability of the surface, that implies that heat transfer is important, because you need a cool surface to condense water from vapor. Beetles do this in the morning, after emerging from underground where it’s warmer than outside,” said King, noting that heat transfer occurs only in the direction of decreasing temperature.

The Namib Desert beetle (genus Stenocara) lives in one of the most arid areas with only 40mm of rain per year, and has developed a unique technique to survive by obtaining water from early morning fog, which is also known as fog basking. It drinks the fog by the means of collecting it on its own bumpy back surface, which provides for accumulation of water droplets.
The Namib Desert beetle (genus Stenocara) lives in one of the most arid areas with only 40mm of rain per year, and has developed a unique technique to survive by obtaining water from early morning fog, which is also known as fog basking. It drinks the fog by the means of collecting it on its own bumpy back surface, which provides for accumulation of water droplets.
With the assistance of 3D-printed spheres (of varying surface textures that resemble the bumps found on the beetle’s wing), a makeshift wind tunnel, and a nebulizer, King is reverse engineering this process to advance his own theory, grounded in physics rather than chemistry. He believes that by altering an object’s shape and texture, it is possible to coax more droplets to collide with, rather than flow around, the object. So far he has found that a sphere with one-millimeter lumps across the surface caught droplets with nearly 2.5 times the efficiency of a smooth sphere with the same surface area.

“It’s basically a filter element,” King said. “For example, you attempt to use your finger to catch fog, the droplets would just go around it. But if your finger had a different shape or texture, maybe it would intercept the water molecules in greater quantity.”
In the Namibian desert and other arid regions around the world, where morning fog is abundant and rainfall is scarce, fog harvesting can be a critical source of water for both humans and wildlife. Leading fog harvesting technology utilizes vertical mesh nets that collect droplets as fog passes through and siphon the water into troughs.
While effective, these nets require a lot of space that may not be available in crowded urban areas. Moreover, with climate change, water droplets in clouds are predicted to shrink, which could mean that mists themselves will get lighter. King’s goal is to figure out how slight variations in shape and texture (rather than fiber diameter and spacing, the parameters studied to improve traditional mesh systems) can filter fog more efficiently under the right wind conditions.
King hopes this research can be used to improve the efficiency of existing mesh systems, and to develop larger installations that utilize building architecture to recreate this effect.
“If you already have some kind of building envelope, by adjusting the shape of that envelope—architectural features on parts of a building—you can change the amount that it’s going to collect fog as it blows by the building," King said. "In certain geographies where you have a lot of fog blowing across the building, modifying the shape of the flow and certain features on the surface could allow you to collect more. Or, say, in a refugee camp, you’ve got a bunch of tents. Maybe by slightly modifying some features on the surface of the tent, you could induce greater collection of water that would run off in the places you want.”
Of the decades of research that have been done on the Namib beetle, King is one of the few—if not the only—scholar to focus on the flow physics of surface morphology.

“There is a lot of money that’s put into researching the condensation in a system like this by changing the wettability. That, we should stop doing,” he said. “It doesn’t seem to follow from the biological example, it doesn’t seem physically relevant, and usually when you change the hydrophobicity of a surface on a large scale, that involves using a lot of toxic stuff.”
King has found inspiration from other structures in nature, such as the mechanical principles behind bird’s nest construction. The elements of the nest, while durable in nature, merge to create something that’s soft and flexible, yet cohesive enough to withstand disturbances. While the research is still in its beginning phases, he believes these findings could provide a blueprint for designing more shock-absorbent structures, built to withstand earthquakes, hurricanes, tornadoes, and other climate change-induced events.

King also sees great potential in his research on termite mounds, and how the tiny insects have managed to build structures that are efficiently ventilated—a challenge that has eluded the most experienced of human architects. He hopes his findings, which explain how the structures regulate heat, humidity, and respiratory gas exchange, will lead to the creation of sustainable, alternative HVAC solutions.

Termite mounds in the far north of Queensland, Australia.
Termite mounds in the far north of Queensland, Australia.
As we seek to mitigate human stresses on the planet—global warming, resource scarcity, environmental degradation, and more—many of these systems in nature have already found solutions, King said. And they have found them without relying on harmful chemicals or extraordinary energy consumption. The tens of millions of species that inhabit this earth, he said, offer a well of tried-and-tested principles for solving design challenges in a more sustainable way.
“Whenever they have a solution, they’re doing it without the cost of energy we use," King said. "The zero-energy idea that we would not necessarily think of…they’re likely to be there. It’s a good place to look.”

Creative Design: Karaamat Abdullah